Recently, I had the opportunity, to listen to Vered Rom-Kedar give a public lecture entitled Billiard is not just a game. Until now, I haven’t thought much about this expanding branch of mathematics but, for me, the lecture highlighted some of the reasons I find mathematics so captivating, and it encouraged me to keep going with my own exploration. In the book Geometry and Billiards, Serge Tabachnikov introduces billiards in this way:
Mathematical billiards describe the motion of a mass point in a domain with elastic reflections from the boundary. Billiards is not a single mathematical theory… it is rather a mathematician’s playground where various methods and approaches are tested and honed. Billiards is indeed a very popular subject…
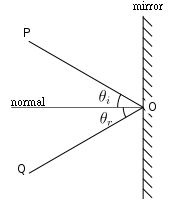
In her public lecture Rom-Kedar started at the beginning. She described the familiar motion of billiard balls as they hit the sides of a billiards table. Once set in motion, a billiard ball will move along a straight line with a constant speed until it hits the side of the table. Its path, after it hits the side, is subject to a familiar law about the reflection of light, specifically, that the angle of incidence equals the angle of reflection. The billiard ball obeys the same law. If it happens to hit the other side head-on it will return to the first side along the same path.
Rom-Kedar then asked the first scientific question: What would happen if the ball just kept moving, traveling in straight lines, as it hit side after side, for an infinitely long time, each time obeying that law of reflection? Will the ball eventually traverse every point on the table? As it turns out, the answer to this question is yes, for some of these events, and no for others. When the ball’s initial move away from the side of the table begins at an angle whose measure has a rational relationship to the dimensions of the table, a periodic orbit gets locked in, and the periodic repetition of paths will never allow the ball to cover all the points on the table. But when the relation between that angle and the dimensions of the table is irrational, the paths are ergodic, i.e. they impinge on all of the points of the given surface or table. These ideal billiards have no mass and hence there is no friction. But in every other way, their behavior is the same as the ordinary billiard ball. I would suggest that there is already something interesting about the correspondence between the rationality of a geometric measure and the action of the ball. Why would there be such a correspondence? It’s like seeing something about numbers through the back of a mirror.
As it turns out, periodic behavior is fairly rare. Ergodic behavior is far more common, There’s a nice narrative about various approaches to this specialization in a 2014 Plus Magazine article by Marianne Freiberger.
In the 1980s mathematicians proved that for the vast majority of initial directions the trajectory will be much wilder: not only will it not retrace its steps, but it will eventually explore the whole of the table, getting arbitrarily close to every point on it. What is more, a typical trajectory will visit each part of the table in equal measure: if you take two regions of the table whose areas are equal, then the trajectory will spend an equal amount of time in both. This behaviour is a consequence of billiards being ergodic. By “vast majority” mathematicians mean that if you pick a direction at random, it will almost certainly behave in this ergodic way.
The absence of a pattern in ergodic behavior makes it very difficult to predict where the ball, or point, might be after some specified amount of time. A computer program could run all the paths fast enough to see what happens, but in true chaotic fashion a very slight change in the direction of the initial trajectory will dramatically change the ball’s later positions. But, as Freiberger explains, because many dynamic physical systems are ergodic, it does give us a handle on something other than the position of a particular point over time. Rather than being able to trace the path of of point.
you can accurately predict what proportion of its time it spends in a certain region of the table. If it’s a gas you are looking at, then you might not be able to say exactly where its many constituent molecules are at any given moment, but you can predict things like its temperature or pressure. So, as chaotic systems go, ergodicity is actually a good thing.
As always happens, mathematicians hunt for all of the generalities associated with all of the imagined, ideal possibilities. And changing the shape of the table introduces a lot of them. Instead of a rectangle, the table could be triangular, hexagonal or L-shaped. It could be round or elliptical. Rom-Kedar said that with these variations, questions about what will happen become “more delicate.” There are many more periodic trajectories in curved figures like circles and ellipses. Most of these events do not explore all of the table.
It is remarkable that billiards models effectively address many phenomena in the physical sciences, that are already described by alternative mathematical models, as well as open questions in mathematics, even number theory. Physicists use it as a close approximation of particle forces and movement. They are relevant to any systems exhibiting chaotic behavior. And, to be clear, billiard models are not restricted to objects on the plane. Billiard models have been developed on various surfaces, including Riemann surfaces.
There is something beautiful about all of this. An observation, of a very specific and pretty limited physical event (a billiard ball on a table) inspires the thoughtful exploration of imagined ideals, that involve infinite times, and are not limited by physicality. These abstractions are a product of looking through the physical consideration to the endless possibilities captured by ideals. Then these thorough investigations of purely idealized possibilities become a way to look at a surprising number of unrelated physical (and mathematical) phenomena. How does the human intellect manage this? And what motivates us to do things like this? It’s beautiful and fascinating.
Fascinating indeed! Even more so when you can play with these trajectories and see th resulting patterns. I made a web page with three “Fluxes” that beg the question of ergodicity.
They’re beautiful. Thank you!